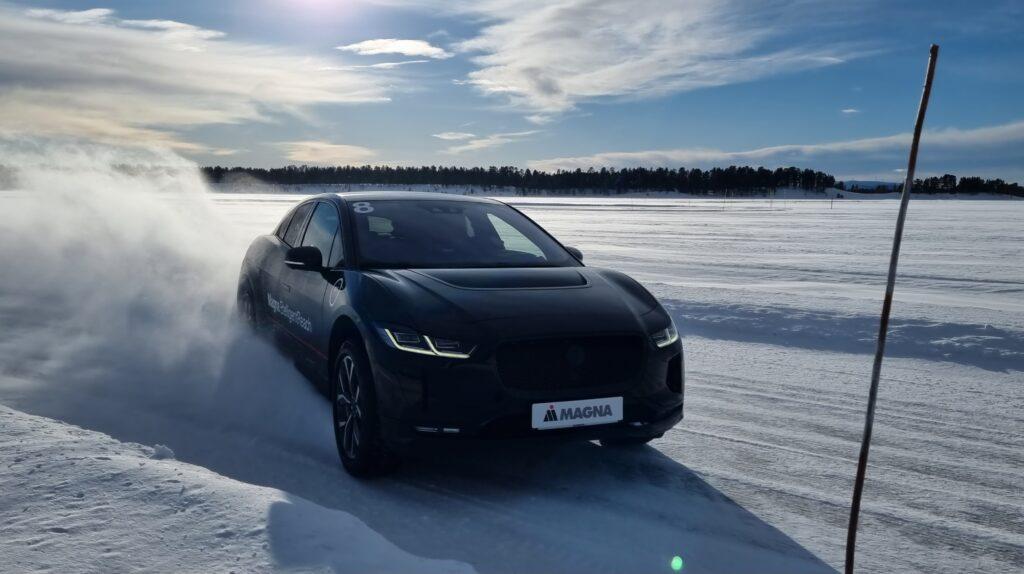
All-wheel drive electrification opens opportunities to improve driving dynamics, safety, efficiency, and convenience. The main levers are choosing the appropriate advanced drive architecture, and consequent functional integration through software.
Magna has been a pioneer in developing and optimizing four-wheel and all-wheel drives, driven by combustion engines. Currently, a shift towards electric all-wheel drives (eAWD) is taking place.
eAWD in BEVs (battery electric vehicles) has a number of advantages: It is easier to integrate, more power is required due to heavy batteries in some vehicle segments, and it enables improved wheel torque distribution. What is more, the drive functions are increasingly defined through software.
Magna improves efficiency, as well as drivability, through the “Magna Vehicle Motion Control approach”.
The Vehicle Motion Control can be seen as the central part of a control loop consisting of five elements: real vehicle input; vehicle physics model; the vehicle motion control itself; the smart actuators it commands; and the resulting real vehicle behavior.
Real Vehicle Input refers to driver actions like steering or operating the accelerator and brake pedal. It utilizes all relevant data provided by onboard sensors like speed, acceleration, and yaw rate. In the future, this information will increasingly be enriched by off-board data.
The car will be able “to look ahead” and add the acquired information.
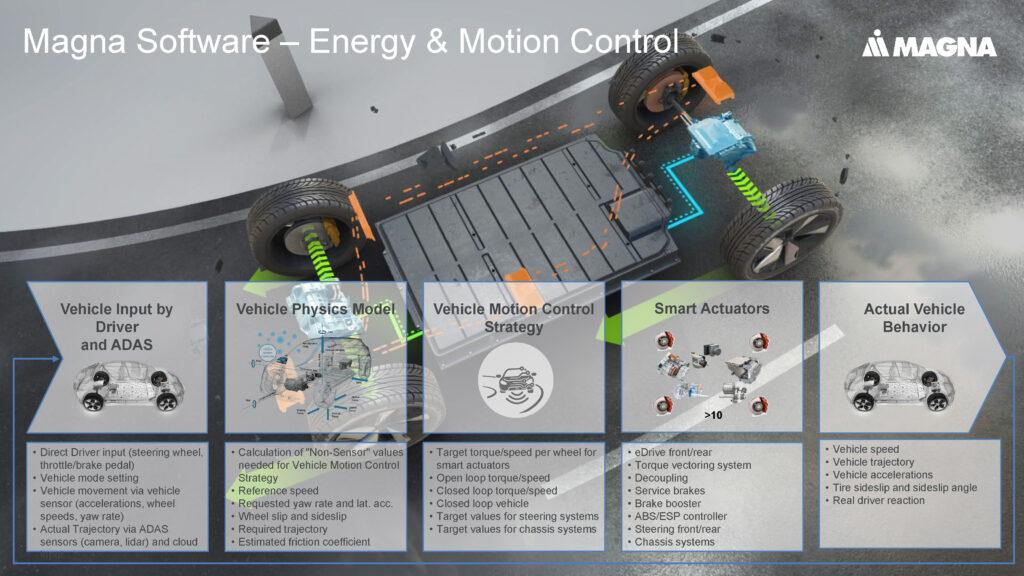
For example, Magna’s future vehicle physics model will contain a feature called “traction predictor”. It will be based on sensor fusion that firstly adds information from cameras, Lidar and other technology, supported by data from the cloud.
The vehicle will watch the road surface ahead and include information from other vehicles which have recently been on the road and share the information gathered through the cloud.
All this data will be combined in the vehicle physics model.
For the vehicle physics model, Magna uses all existing information that the vehicle and the drivetrain provide.
With this data, the vehicle will be modeled as a “virtual twin”. This enables the system to calculate how the vehicle moves, or how it will be positioned on the upcoming route.
Crucial aspects are what is known as side slip calculation and the related active sideslip angle limitation.
The sideslip angle describes how much the axles slip sideward in relation to the intended trajectory, leading to over- or understeering. In current state-of-the-art vehicles, closed-loop control is mainly done by comparing the actual yaw rate to the requested yaw rate and the actual accelerations to the requested one.
Magna has worked on additional input, enabling it to realistically calculate the sideslip on both axles.
Step three in the loop is the vehicle motion control strategy. This is the onboard software that enables the vehicle to follow the targeted trajectory. It controls all relevant actuators like the e-drives, wheel brakes, possibly clutches, and active chassis components.
For example, it will split the torque between both axles to increase driving stability and provide a certain mode setting to individualize the vehicle behavior. These modes can range from a very stable standard setting to sporty modes with a certain amount of controlled drifting.
In the near future, the motion control software will optionally be able to control active suspension components, active rear wheel steering, and include the management of steer-by-wire systems.
Smart Actuators are the next element in the control loop. Besides the wheel brakes, torque vectoring elements, brake hydraulics, brake booster, and decoupling devices. Magna also defines the e-motors as smart actuators. This is because the performance of e-motors in the system is a crucial element to manage the torque between the front and rear.
The motors can take over a large part of the wheel brake tasks in many driving situations. Magna is integrating the e-motors and brakes into one single domain. The fifth step, the real vehicle behavior, is described through an example below.
To place it in context, Magna has over the past few years developed several e-drive configurations that enable lateral torque vectoring in different ways. These include systems, where two e-motors are coaxially positioned on the axle.
They include drives that utilize a superpositioning motor to shift the torque between left and right.
Another approach is using one motor with two clutches for lateral torque vectoring. Magna also offers a system that integrates the wheel brake function in such a system by merging both functional domains. This allows for advanced vectoring functionality without any physical lateral torque vectoring elements.
“Merging” practically means that two domains are integrated into a single new domain.
The vehicle motion controller will command the torque and speed request for each wheel prioritized and include single wheel brakes when necessary. So, there is no longer a need for two vehicle physics models for the related controllers.
Vehicle behavior
In the following, an example is given of how different vehicle configurations will behave on the road. We compare an all-wheel drive vehicle without torque vectoring with an eAWD vehicle including integrated control of e-drive and brake. Both vehicles are driven on the same track by the same driver at the same speed. The image captured from a video shows a typical situation.
The tire slip indicator in the bottom right shows how each wheel behaves in a certain driving situation. The circle of the wheel slip indicator essentially represents the friction value that may not be exceeded to maintain driving stability. Each dot represents one of the four wheels.
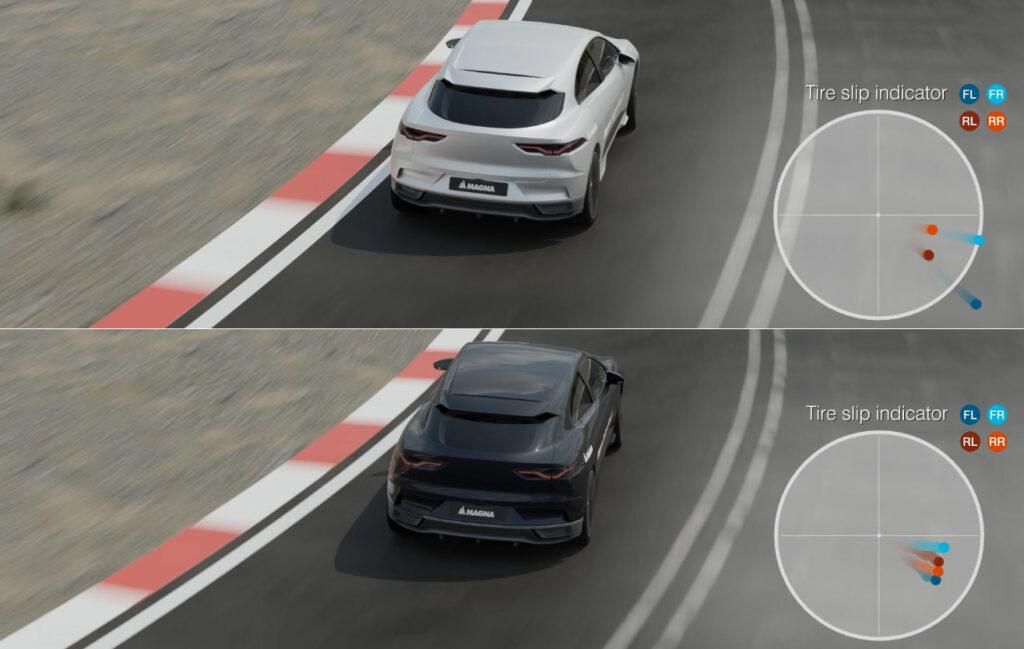
The size of the circle represents the given friction value. In this example, the circle has a constant size during driving, because the friction value remains constant on the track. With a reduced friction value, the circle would be smaller.
This illustrates, why the combination of the control loop steps described above, and the central role of the motion control software is crucial.
In the driving situation shown, the eAWD vehicle with the integrated control of e-drive and brake (black) is stable, while the standard eAWD vehicle (white) exceeds the traction limits with its front wheels. The control software manages to keep the “stress” of each wheel on an almost equal level. While the driver of the white vehicle needs to expend a lot of effort to keep the vehicle on the road, the black vehicle remains stable and has a considerable safety margin.
The improved controllability enables several advantages: The efficiency benefits, because of improved brake blending and a higher regeneration share through the e-motor allowing for reduced power consumption. This especially applies to real-world driving, due to generally higher longitudinal accelerations than in current homologation driving cycles.
As to safety, the trajectory for every driving situation is significantly improved.
It is easier for the driver to handle the vehicle even in critical situations like avoidance maneuvers, low friction spots etc. This also applies to regeneration and braking, as the integrated software domain allows for better controllability.
The vehicle dynamics benefit from an increased achievable lateral acceleration and the related increase in cornering speed dynamics within the physical limits.
Finally, the improved controllability such as reduced steering effort, as well as the predictive abilities allow for clearly improved comfort and convenience.
More Stories
Automotive Industries interview with Johannes Mark, Head of Global Parking Insights, EasyPark Group – Transforming Urban Parking with Light Detection and Ranging Technology
How Secure Parking Technology is Shaping the Future of Urban Development
Click Shield from MIKROE simplifies integration of Arduino Portenta with over 1600 Click boards