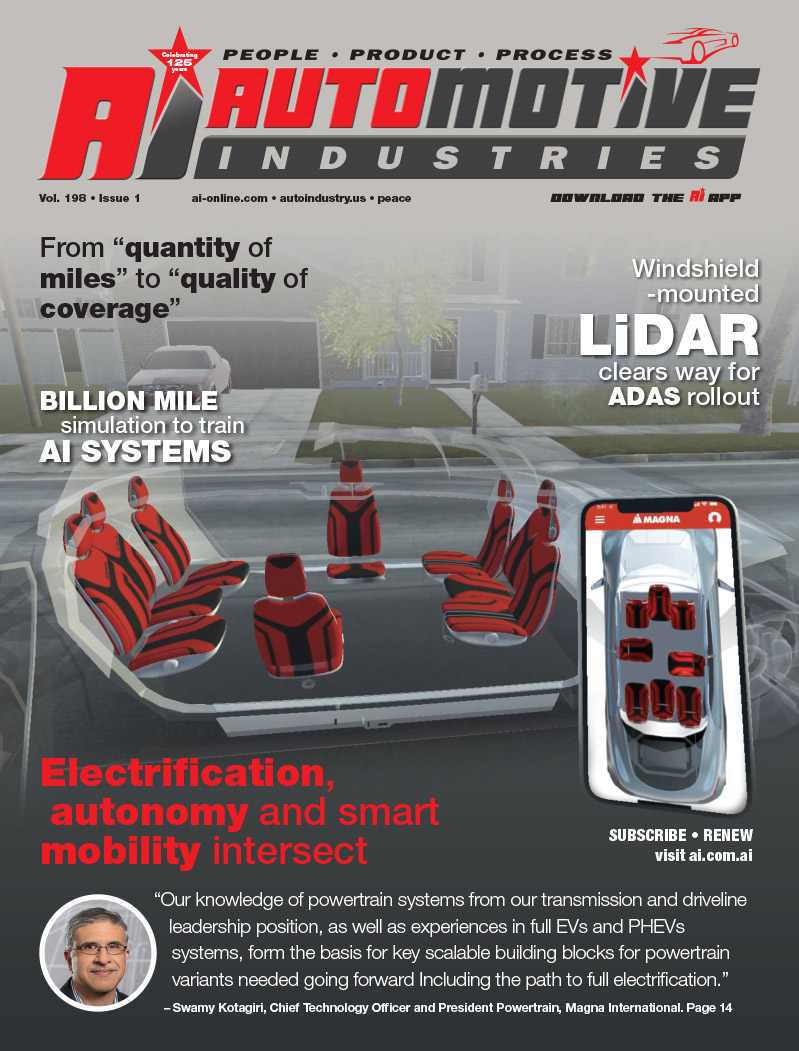
We hear a lot about HEVs and more people are getting involved from the designers to the users. The designers are in their world and provide the necessary response to a demand for transportation that doesn’t rely upon the dwindling source of petrol and contaminates the environment. Users get their technical information from user guides sold with the vehicle, and, for the most part, that is sufficient for them to give basic care. However, the most critical link in the chain for keeping that vehicle on the road is the technician. There are two basic groups, the supervising technicians who have more of a formalized training knowledge in maintaining the vehicle according to the designer’s specification and the general technician who performs the mechanical and day by day maintenance and who may work in a general repair shop. Documentation is needed between the designer and the consumer that provides that maintenance information. However she or he should be able to explain the “why” of a procedure. Numerous brands of vehicles are emerging, and it is difficult, if not impossible to keep in mind the specifications for all vehicles. Keep in mind, too, that HEVs still are not the only vehicles on the road. Yet, they demand special consideration, and documentation also needs to be in the forefront. What the technician sees are repair manuals, such as a Mitchell, Chilton, Motor, or Haynes, and they are very specific, often with little theory or the “why” behind what is being done. Literally, the procedures are mechanical, and if there an exceptional situation, there is no instruction on how to manage it. It is the understanding of the theory of operation of a vehicle and the ability to apply it that makes for an excellent technician. Auto mechanics is not like it used to be, where someone could drop out of high school and turn a wrench under a tree. With today’s sophistication, the technician usually winds up getting certification after going through a community college or very sophisticated vocational school, taking courses in electronics, computers, and high-level automotive technology. Automotive technology no longer is easy.
This document is an example of the level of knowledge that a competent supervisory automotive technician should have either to do repairs or direct others to do them. It also serves the function of informing those with more of a scientific bent of not only what is involved in HEV maintenance, but the reasons behind it. The technician learns how thermodynamic theory is applied to HEV in an effective way in an in depth way close as possible short of actual design. In the same breath, the academic style of footnoting talks “up” to the technician and not “down”, thus conveying an atmosphere of respect. The references emphasize the importance of knowing from where information is derived, showing that one must be able to provide evidence for assertions and actions. They are part of the vital critical thinking processes underscoring a modern automotive technician’s approach to problem solving. It also is written for the purpose of informing designers of the practical issues of getting technicians to understanding the intent of the HEV design. This is not meant to be a comprehensive document but it can serve as a basis upon which to build a more complete presentation of thermal management in HEVs. It is more about the form of knowledge than complete content. So, here goes about what might be in that technician’s guide or minicourse on HEVs.
HEVs in general
A Hybrid Electric Vehicle (HEV) is one in which a combination of a liquid hydrocarbon powered engine and electric motor are used for propulsion. These vehicles are being developed by most major car manufacturers in response to the realization that there will be less petroleum in the future. Reserves are declining and the world be facing what is known as “peak oil”, where the rate of petroleum discovery will be less than the rate of consumption. At some point, there is the real possibility that people will have to rely upon electricity as the sole source of vehicle propulsion. This is to say nothing about the second greatest source of air pollution coming from vehicles [1]. For now, however, we need to consider HEVs.
The integrity of an HEV depends upon the integrity of two of the most critical systems, the electric power generation and storage system as well as the thermal control system. It is essential that one understands major principles underscoring the operation of these systems and maintains them properly. There are many types and designs of HEVs, so it is more productive to consider the general thermodynamic principles governing heat and power generation and the means available for thermal regulation. Different vehicles will have different configurations, so it is impractical to direct our attention to specific cases. However, our modularized approach, starting with a discussion of theory, components, and system types will allow appropriate application to specific cases.
We have here only a survey of what is involved in considering thermal regulation in HEVs. A detailed coverage would involve numerous courses in thermodynamics, mechanical design, and mathematics, to name a few areas. This document is directed to well trained HEV supervisory technicians but there are parts that anyone all the way down the repair chain, including ordinary drivers can derive benefit.
It may be noted here that one can get confused or misdirected by over complicating matters. Often, dropping back a few paces and re-examining fundamental principles will lead one directly to a solution. This does not trivialized thermodynamics, as calculation of heat generation and flow can be just as complicated as nuclear physics, as just about any meteorologist will testify. Modeling is very complicated.
“Vehicle” means cars, trucks, and any other means of conveyance that is self-propelled and using electric or a combination of fossil fuel-electric combination of energy source, although this article does not make them our focus, nevertheless, our discussion will apply to motorcycles and scooters.
Several types of HEV exist:
 Plug-ins
 Fuel Cell-based
 Gas/diesel-Electric hybrids
Plug-ins
Inasmuch as plug-in vehicles don’t use gas and rely solely upon battery power, the battery packs, they are much larger than those found in gasoline-electric hybrids. The greater the range one wants the vehicle to have, the greater size the battery pack will be, and more weight, the greater stress there will be on each moving component of that vehicle, due to friction.
Fuel Cell-based HEV
Fuel cells are a special type of battery, and fuel cell HEVs run both with a hydrocarbon-fueled motor (gas, diesel, or other hydrocarbon) motor and an electric motor. The fuel tank contains the liquid fuel for the hydrocarbon-fueled motor, and the fuel cell is the energy supply for the electric motor.
Gas/Diesel-Electric hybrids
The hydrocarbon part of the car is a conventional system, except that it is linked to the electrically powered one. The electric system is powered by a battery, and that is charged either by an alternator running off the gasoline engine or by a wall charger, or a combination of both, as in a plug-in hybrid vehicle (PHV). Regardless of the system, thermal control is an issue, although it is more of a problem in gasoline-based engines and vehicles carrying heavy loads than in other cars.
Review of general thermodynamic theory
Before dashing off to read about particulars of systems, it is best to think about an algorithmic approach to heat transfer and regulation issues in HEVs – or anything else, for that matter. We establish some general principles and theories and then apply them. In particular it is useful to review how heat transfer is affected by materials and environment. Then, we can apply this knowledge to particular situations in controlling it.
Heat and its sources
Heat, itself is one of the five types of energy, the others being mechanical, chemical, nuclear, electrical, and. Heat is the motion of molecules or atoms. Mechanical energy is defined as work, the sum of force in a specific direction [2]. Chemical energy is that which results from the changes in the associations of atoms in molecules that are bonded together. Nuclear energy comes from forces within the atom. Electrical energy is the flow of electrons.
First, we must know the difference between heat and temperature. Heat, simply put, is the energy created by the movement of atoms and molecules. The only situation in which there is no heat is when neither atoms nor molecules move. However, this is only a theoretical situation, as atoms always are in a constant state of motion, even in the depths of space. Thermal images will confirm this [3]. Temperature is the measurement of average heat in a space or thing. The heat in a small steel ball bearing of two mm in diameter may be the same as that of the Empire State building; size is irrelevant. In theory, one measures the heat of each molecule, sums it, and divides by the number of molecules to get the temperature. The average of 1645/35 = 47 = 141/3, meaning if the total heat content of the first is 35 and there are 1645 molecules, the temperature would be 47. For the second situation, if the total heat content is measured is 141 and there are three molecules, the temperature still is 47. Heat results from the speed of the molecules, which results in more energy. However, we must consider why they move. Energy is not self-generated. Something imparts energy to the molecules, and this comes from the atoms, themselves.
Temperature is measured in three ways: Fahrenheit, Celsius, and Kelvin. A quick glance at SI units is in order. “SI units” means “International System” [4]. We say “SI”, as this is an abbreviation for the French, Le Système International d’Unités [5]. Note that the conversion formula between Fahrenheit and Celsius is: C/F-32 = 5/9.
Heat energy is measured in Joules, named after James Prescott Joule (1818–1889), an English physicist. Other units of heat are the British Thermal Unit (BTU) and the calorie. The former is known by dieticians and weight watchers, and the latter, BTUs, are familiar to those installing thermal control systems. However, the scientific community talks more in terms of joules, and this is the units that we will discuss at present.
Joules
As defined in the SI: J = kg2 • m2/s2 = N • m = Pa • m3 = W • s,
where J is joule, kg is the kilogram, s is the second, N is the newton, m is the metre, Pa is the pascal, and W is the watt.
Watts is how fast energy is transferred. That is: (W)atts = (J)oules/(S)econds, W=J/S It is the energy required to create one watt per one second, otherwise known as “watt-second”. Wikipedia gives some excellent practical examples, as it is hard to picture what these equations mean in reality.
One joule in everyday life is approximately:
 the energy required to lift a small apple one metre straight up. (A mass of about 102 g = 1⁄9.81 kg)
 the energy released when that same apple falls one metre to the ground.
 the energy released as heat by a person at rest, every hundredth of a second.
 the kinetic energy of a 50 kg human moving very slowly (0.2 m/s).
 the kinetic energy of a tennis ball moving at 23 km/h (14 mph) [6].
British Thermal Unit (BTU)
The BTU is about the same as 1,055.05585 joules. In more graphic terms, it is the amount of energy required to raise the temperature of one pound of pure water (0.454 kg) from 39°F (3.9°C) to 40°F (4.4°C). As with persons using the more efficient metric system, they have been getting away from the BTU and using the joule.
Calorie
While the term is archaic – going back to the one who coined it, Nicolas Clément in 1824 – dieticians evidently don’t think so. It, too, has been replaced by the joule. There are two types of calorie: small, or gram; and, large, otherwise known as kilogram calorie, dietary calorie, or food calorie (Cal). The small calorie is the energy needed to raise the temperature of one gram of pure water by one degree centigrade, or approximately 4.2 joules. The large calorie is equivalent to the energy required for raising the temperature of one kilogram of pure water by one degree centigrade. One large calorie is equal to 1,000 small calories, approximately 42,000 kilojoules.
Heat transfer considerations
Three modes of heat transfer need to be investigated in our consideration of thermal management in HEVs: conduction, convection, and radiation. We need to know the source(s) of each of these three types and to regulate them. Knowing this information will help in tracing the sources of heat and the means by which heat travels.
Conduction
Conduction literally is the travel of molecular energy within a solid or liquid. This is relevant to our discussion of thermal management in considering materials out of which parts are made. For example, aluminum heads in an engine are more susceptible to changes from heat differences than cast iron ones. Hence, greater attention needs to be paid to their care and management.
Convection
Simply put, convection is molecular movement within fluids, including liquids and gasses. Technically solids are fluids, but we don’t think of them as such, as the molecules move ever so much more slowly. Yet, geologists think of solids as fluids, especially when considering geologic processes over very long periods of time. Individual particles move randomly through a fluid and currents within the fluid will transport molecules. The first is called “Brownian movement”, and, for all intents and purposes is random, i.e., there is no way to predict where a particle will be precisely at any given moment. Advection, the second process of molecular transport, happens on a larger scale. In thermal management, one must pay attention to areas in which heat may circulate such in confined spaces. Batteries that sit in an enclosed area without ventilation may be susceptible to damage, because there is no air flow to transport the heat generated by electrochemical processes in those batteries.
Radiation
Particles traveling through space are energized, just by virtue of the fact that they move. Hence, heat is involved. That they move through space means that the heat travels by radiation. In a uniform space, heat travels in all directions equally from that heat source. Electromagnetic radiation, then, creates heat radiation. Engineers need to pay particular attention to black body radiation, which is an ideal radiator that emits or absorbs the maximum amount of radiation possible for that body. A black body does not reflect any energy, but, it, itself, is whatever emits or absorbs that energy. The intensity of radiation is measured in terms of power (watts) per unit area and we get into some rather interesting physics at the sub-atomic level concerning the smallest of the smallest, or Planck volume (4.22419 × 10−105 m3 ). Black body radiation is important in considering what a material will do when sitting in a particular temperature environment. If it is hotter than the ambient temperature, it will radiate heat. If it is colder, it will absorb it. This common sense view helps us in assessing how to place heat sinks as thermal evening devices and what color to make them. As a general rule, darker colored bodies absorb and radiate more than lighter colored ones, the latter also being more reflective. Black cloth absorbs heat; light cloth reflects it. Persons designing solar products use this black body property, but it also applies to HEV design, as well in thermal management.
Once we have identified sources of heat and how heat travels generally, we may start focusing on thermal regulation. Of course, one may have high heat, accompanied by a high temperature, and low temperature and low heat content. Nevertheless, the first sign of heat change is indicated by a measurement of temperature. If there is a transference or generation of heat within an environment, the temperature will rise.
Various factors affect heat transfer in a substance, such as:
 Material composition of objects
 Type of heat
 Environment
 Intensity of heat source
 Ability of objects to absorb heat
 Color of objects.
How fast heat travels – the rate – is called “heat flow”, or heat flux, and the symbol for this is Q. The unit measurement is in watts, or W. This is the same unit as used in electricity and often is referred to in terms of power. In essence, it means how much energy is transferred per unit time.
We have heat flow per unit area (A), itself, also called “heat flux”, designated by the letter “q”. We now can state that q=Q/A. However, since watts (W) is the unit measurement for Q (heat flux), we have: (W)atts per (m)eter squared, or W=W/m2. Now, we consider the material involved in heat transfer.
Volume is thickness multiplied by area, and we need to consider the temperature difference between the substance and its environment (including adjoining substances), and the material, itself.
Further information, including the full conference program, articles and whitepapers are available on
More Stories
How Modern Power Management Enhances Connected Fleet Tech
Automotive Industries interviews Bahar Sadeghi, Technical Director, Car Connectivity Consortium (CCC)
Avery Dennison PSA tapes support rapid evolution of EV batteries